Translate this page into:
Genetic diagnosis of skeletal dysplasias causing short stature in children
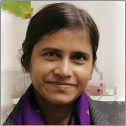
*Corresponding author: Inusha Panigrahi, Genetic Metabolic Unit, Department of Pediatrics, Advanced Pediatric Centre, Post Graduate Institute of Medical Education and Research, Chandigarh, India. inupan@yahoo.com
-
Received: ,
Accepted: ,
How to cite this article: Panigrahi I, Daniel R, Kaur K. Genetic diagnosis of skeletal dysplasias causing short stature in children. J Pediatr Endocrinol Diabetes 2023;3:9-14.
Abstract
Short stature may often be due to skeletal dysplasias affecting the limbs, spine, or both. A careful clinical evaluation will help in identifying the rhizomelic and mesomelic shortening of the limbs and scoliosis. The mutations in different genes involved in various pathways in skeletal development lead to phenotypes that present from infancy to childhood or adulthood. A systematic clinical evaluation with identification of the limb bowing or deformity, dysmorphic features, radiological findings from skull to toes, and a single gene or multi-gene panel testing will help in making an appropriate diagnosis. The clinical clues to skeletal dysplasia include skeletal disproportion, unexplained limb bowing, recurrent fractures, facial dysmorphism including flat facies and blue sclera in severe cases, and sometimes typical digital or cardiac abnormalities. The following review focuses on the postnatal presentation of skeletal dysplasias mostly referred for evaluation of short stature.
Keywords
Bone disorders
Deformities
FGF signaling
Growth retardation
Skeletal disorders
INTRODUCTION
Skeletal dysplasias are a heterogeneous group of genetic disorders with abnormalities in different pathways and genes involved in bone development, mineralization, and differentiation. The signaling pathways implicated include the Wingless and Int-1 (WNT) signaling, NOTCH signaling, fibroblast growth factor (FGF) signaling, and Hedgehog signaling pathways.[1,2] The mutations in different genes involved in various pathways lead to phenotypes that may present from infancy to childhood or adulthood. The lethal skeletal dysplasias represent the most severe phenotypes of skeletal dysplasias involving poor formation, growth, and mineralization of limbs, and thorax and/or skull bones.[3] The latest nosology for skeletal disorders published in 2023 lists the different groups of these disorders with genes involved and the OMIM identification numbers and includes over 750 entities.[1] However, the field is evolving and with the addition of more types of skeletal dysplasias, it is becoming more and more difficult to make an appropriate diagnosis of a rare or an ultra-rare disorder. The following write-up summarizes the different disorders commonly encountered in clinical practice and provides an approach to their diagnosis and management. In patients with short stature, skeletal dysplasias accounted for around 11% in a study from a developing country, the most common being achondroplasia (ACH) and mucopolysaccharidosis (MPS).[4]
Initially, the different skeletal dysplasias were clinically classified according to the part of the skeleton involved, the age of onset, and the clinical severity. Here, we focus more on skeletal disorders with limb involvement. Conventionally, they were mainly divided into disorders with rhizomelic limb shortening or mesomelic or acromelic limb involvement [Table 1]. Some disorders present with vertebrospinal involvement and trunk shortening. Furthermore, based on primary epiphyseal, metaphyseal, or spinal affection, some disorders include multiple epiphyseal dysplasias (MED) or different metaphyseal dysplasias which can present with a rickets-like phenotype. Some skeletal disorders may also have spondyloepimetaphyseal or spondyloepiphyseal involvement [Table 2].[1,5]
Rhizomelic SKD | Mesomelic SKD | Acromelic SKD |
---|---|---|
1. Achondroplasia 2. Rhizomelic chondrodysplasia punctata 3. Spondyloepimetaphyseal dysplasia – Aggrecan type 4. LBR-related rhizomelic skeletal dysplasia 5. Spondyloepimetaphyseal dysplasia with rhizomelia – Borochowitz Cormier Daire (AR) type |
1. Langer mesomelic dysplasia 2. Robinow syndrome 3. Leri-Weill dyschondrosteosis (SHOX, SHOXY) 4. SLOS 5. Mesomelia-synostosis syndrome 6. Mesomelic dysplasia – Kantaputra type 7. EN1-related Endove syndrome |
1. Acrodysostosis 2. Chondroectodermal dysplasia (Ellis-van Creveld syndrome) 3. Angel shaped skeletal dysplasia 4. Geleophysic dysplasia 5. Acromicric dysplasia |
SKD: Skeletal dysplasia, SLOS: Smith-Lemli-Opitz syndrome, SEMD: Spondyloepimetaphyseal dysplasia, AR: Autosomal recessive
Spondyloepiphyseal dysplasia | Spondyloepimetaphyseal dysplasia | Other skeletal dysplasias |
---|---|---|
• SED congenita (AD) | • SEMD, short limb hand type (AR) | • Omodysplasia (AR) |
• Progressive pseudo-rheumatoid dysplasia (AR) |
• SEMD with joint laxity (AD, AR) | • Chondrodysplasia with joint dislocations (AR) |
• SED with joint dislocations (AR) | • SEMD, X-linked | • Multiple joint dislocations with short stature (AD) |
• SED Maroteaux type (AD) | • SEMD with craniosynostosis, Faden-Alkuraya type (AR) | • Stickler syndrome (AD, AR) |
• SED Stanescu type (AD) | • SEMD, Sponastrime type (AR) | • Joint dislocations with or without ID (AR) |
• SED tarda (X-linked) |
AD: Autosomal dominant, AR: Autosomal recessive, ID: Intellectual disability, SED: Spondyloepiphyseal dysplasia, SEMD: Spondyloepimetaphyseal dysplasia
DIAGNOSIS
When should a pediatric endocrinologist consider the possibility of skeletal dysplasia when evaluating a child with short stature?
The main clues to the diagnosis of skeletal dysplasia in a child brought with short stature include the presence of disproportion, multiple fractures detected clinically or on X-rays, unresponsiveness to calcium and vitamin D therapy in a child with rickets, and X-rays showing significant metaphyseal or epiphyseal abnormalities or the presence of dislocations. The presence of additional cardiac, digital, or ear abnormalities may point to an underlying syndrome.
What are the common forms of skeletal dysplasia presenting with short stature?
The most common skeletal dysplasias usually seen in a pediatric clinic include ACH, mucopolysaccharidoses, and spondyloepiphyseal dysplasias (SEDs). Other causes of short stature such as celiac disease, hypothyroidism, and chronic renal disease should be ruled out by performing appropriate tests.
What are the steps in making a diagnosis and genetic testing of skeletal dysplasias?
Although some skeletal and joint involvements have variable phenotypes, most of the commoner ones present with short stature.[6,7] The initial clinical evaluation includes finding any disproportion which involves measuring limb lengths and the upper segment to the lower segment ratio. The radiological survey for skeletal dysplasia involves doing X-rays of the upper limbs, lower limbs, pelvis, spine, and hands (including wrist) to look for the type of involvement – rhizomelic, mesomelic or acromelic; or if there are additional defects in the spine.
The confirmation of the diagnosis would require biochemical and molecular evaluation in most cases apart from radiological characterization. The biochemical tests include serum calcium, phosphorus, and alkaline phosphatase levels. Urinary biomarkers such as pyridinolines or collagen breakdown products are tested in some specific cases. Bone-specific alkaline phosphatase can also be done in some selected cases. Enzyme testing on heparinized blood samples confirms the type of MPS in most cases. Deoxyribonucleic acid testing may be needed in some cases to confirm the diagnosis if enzyme testing is not available or shows borderline values. [Table 3] lists the common disorders encountered in pediatric practice. Figure 1 shows bowing seen in osteogenesis imperfecta (OI) and metaphyseal dysplasia, and Figure 2 depicts the phenotypic presentation in hereditary multiple exostosis. Dysostosis seen in MPS patients is shown in Figure 3.
Condition | Major features | Genes involved |
---|---|---|
1. Achondroplasia (AD, sporadic) |
Macrocephaly, rhizomelic limb shortening, short stature, trident hands | FGFR3 |
2. Osteogenesis imperfecta (AD, AR) | Recurrent fractures, bowing of bones, short stature, hearing loss, joint laxity, and rarely brain involvement | COL1A1, COL1A2, CRTAP, WNT1, LEPRE1, SERPINF1, SERPINH1, CREB3L1, PLOD2 |
3. Hereditary multiple exostosis (AD) |
Bony swellings over limbs/joints, exostosis on X-rays, short stature, cervical myelopathy, and coxa-vara | EXT1, EXT2 |
4. Cleidocranial dysplasia (AD) | Short stature, flat facies, dental anomalies hypoplastic/aplastic clavicles, and wormian bones | RUNX2, CBFB, MSX2 |
5. Mucopolysaccharidosis (AR, XLR) |
Short stature, coarse facies, recurrent respiratory infections, cardiomyopathy, seizures, behavioral issues, and dysostosis | IDUA, IDS, ARSK, GLB1, HYAL1, ARSB, GUSB, GALNS, NAGLU |
6. Spondylometaphyseal dysplasias (AD, AR) | Short stature, metaphyseal widening, platyspondyly, and irregular metaphyses | TRPV4, PAM16, ACPS, GPX4, CFAP410 |
7. Pycnodysostosis (AR) | Short stature, dental anomalies, dense bones, and osteolysis of distal phalanges | CTSK |
8. Multiple epiphyseal dysplasia (AD, AR) | Club foot, arthralgia, scoliosis, hip dysplasia, small epiphyses, and flat proximal femoral epiphyses | COL9A1, COL9A2, COL9A3, MATN3, COMP, CANT1, SLC26A2 |
AD: Autosomal dominant, AR: Autosomal recessive, XLR: X-linked recessive, FGFR3: Fibroblast growth factor receptor 3 gene

- Radiograph showing osteopenia, fractures, and bowing in osteogenesis imperfecta (a) and bowing of femur and metaphyseal flaring and irregularities in Schmid metaphyseal dysplasia (b).

- The swellings over the lower limb (a) and X-ray showing bony projections or osteochondromas at the upper end of the tibia and lower ends of the femur (b) in hereditary multiple exostosis.

- Dysostosis seen on X-rays in mucopolysaccharidosis (MPS). There is kyphosis, anterior beaking and platyspondyly in MPS IV type A (a) and shortening, irregularity, and proximal pointing of metacarpals in hand X-ray in the same patient (b).
A simple and practical approach to the genetic diagnosis is illustrated in the flowchart [Figure 4].

- Flowchart depicting the clinical approach to genetic testing in skeletal dysplasias.
Is there a phenotype-genotype correlation in the clinical presentations?
There is significant phenotypic variability in the clinical presentations, though the gene involved may be the same. This is known as phenotypic heterogeneity of that gene-related skeletal disorder. Two examples include the fibroblast growth factor receptor 3 gene (FGFR3) and collagen type 2 alfa 1 (COL2A1) gene.
The phenotypes related to the FGFR3 gene include ACH, a common skeletal dysplasia causing short stature, thanatophoric dysplasia, which is a lethal skeletal dysplasia and CATSHL syndrome in which there is tall stature along with camptodactyly, scoliosis, and hearing loss.[8] In the SADDAN phenotype, there is severe ACH, along with developmental delay and acanthosis nigricans, and early respiratory failure leads to mortality. Thus, thanatophoric dysplasia, ACH, and hypochondroplasia (HCH) are all the result of pathogenic variants in the FGFR3. ACH can be easily diagnosed by Sanger sequencing. The two common mutations FGFR3:c.1138G >A and c.1138G >C in this gene account for 98% of patients with ACH leading to p.Gly380Arg change in the protein. In SADDAN, there is a different mutation, c.1949A >T.
In the case of COL2A1 also, there are variable phenotypes, as shown in Figure 5. Over 700 variants causative of the phenotype have been reported in the COL2A1 gene, which is a large gene with 54 exons. These clinical phenotypes of type II collagenopathies include MED, Stanescu type SED, spondyloepimetaphyseal dysplasia, spondyloperipheral dysplasia, and Kniest dysplasia.[9] The characteristic features include myopia, hearing loss, and cleft palate in addition to short stature. Glycine substitutions in the amino acid sequence of the protein account for over 60% of cases.[9] In phenotypes involving large genes or multiple genes, like OI, high throughput sequencing technologies can be used.[10] With the aid of massively parallel sequencing technologies, the entire exome (whole-exome sequencing) or specific exome regions (clinical exome sequencing and panel analysis) can be quickly sequenced in a single assay on the blood sample of a singular patient.[11,12]

- The phenotypes reported with pathogenic and likely pathogenic variants in the COL2A1 gene. Appropriate diagnosis requires detailed clinical and radiological evaluation.
CASE SCENARIO
A 4-year-old male child presented with short stature, recurrent respiratory infections, and anal abnormality for which he had undergone surgery. Examination revealed flat facies, prominent eyes, depressed nasal bridge [Figure 6], mesomelic shortening of limbs, and microphallus. Routine dysmorphology evaluation was done to look for additional vertebral, cardiac, and/or renal anomalies. Few hemivertebrae were observed on X-rays of the dorsolumbar spine and lower limb X-rays showed mesomelic shortening of lower limbs. A possibility of Robinow syndrome was kept and targeted next-generation sequencing was performed. Two likely pathogenic variants were identified in the exon 9 of the ROR2 gene in the child. These mutations were ROR2:c.2206 C>T (p.Arg736Trp) and ROR2:c.1969 C>T (p.Arg657Cys) confirming the diagnosis of Robinow syndrome. This syndrome should be suspected in patients with upper respiratory problems, as there is subglottic stenosis in these patients. They also present with short stature and mesomelic or acro-mesomelic shortening of the limbs.[13] Short stature, short nose, and brachydactyly are seen in over 90% of patients.

- Robinow syndrome: Photograph showing flat facies, prominent eyes, and depressed nasal bridge.
MANAGEMENT
What are the therapeutic strategies for skeletal dysplasias?
The cornerstone of the treatment in OI includes the optimal management of fractures and the administration of bisphosphonates. These can be given by intravenous (IV) administration as for zoledronate and pamidronate or orally as for risedronate and neridronate therapy.[10] Newer treatments on the horizon include denosumab and recombinant parathyroid hormone (PTH) but these are unlikely to be of significant benefit unless given in combination with bisphosphonates.
Similarly, there is another antibody being studied in OI – fresolimumab, which acts through transforming growth factor-beta inhibition. Anti-sclerostin therapy is also under consideration in OI and it leads to inhibition of the WNT-beta-catenin signaling pathway.[14] It has been found to be promising in mouse models of OI. Another form of therapy under trial is an infusion of allogeneic fetal-tissue-derived mesenchymal stem cells prenatally and/or postnatally to improve bone strength (European multicentric trial).
There are conflicting results on growth hormone therapy in ACH and HCH. A newer drug that has been approved for the treatment of ACH is vosoritide, a recombinant C-type natriuretic peptide analog. It has been approved by the Food and Drug Administration of the USA for children above 5 years of age and also by the European Medicines Agency. Administration of this drug can increase the growth of the child with ACH by 1–1.5 cm/year.[14]
Other agents being tried include soluble forms of FGFR3, meclizine, and PTH for improving longitudinal skeletal growth.[15] Infigratinib inhibits phosphorylation of FGFR3 by selective tyrosine kinase inhibition and is undergoing trials in patients of 3–11 years of age.[16]
Enzyme replacement therapy (ERT) is the mainstay of therapy in many forms of mucopolysaccharidoses such as MPS I, MPS II, and MPS VI. The predominant skeletal form of MPS is MPS type IV and it is of two subtypes – type A and type B. In MPS IV type A, ERT is approved and available from Biomarin Pharmaceuticals, though quite expensive.[17] If financial support is available from the Government through the rare disease treatment policy or other channels for reimbursement of financial support, then this can be tried for some selected patients so that subsequent cardiopulmonary complications can be prevented.
Additional drugs used recently in different disorders with promising results include palovarotene in fibrodysplasia ossificans progressiva, burosumab in X-linked hypophosphatemic rickets, and asfotase alfa in hypophosphatasia.[15,18] Palovarotene binds to retinoic acid receptor gamma as an agonist and blocks BMP and SMAD signaling pathways. Asfotase-alfa carries the active site of the tissue’s non-specific alkaline phosphatase; it acts as a replacement therapy with a selective role in bone mineralization. Furthermore, resveratrol is being tried in pseudo-ACH. It decreases the acetylation of SOD2 and ROS and also activates the AMPK pathway. N-acetyl-cysteine has been recently used in dystrophic dysplasia and found to be effective in mouse models.
Is prenatal diagnosis possible? How should we go about genetic counseling?
In severe phenotypes of skeletal disorders such as recurrent fractures and multiple dislocations, prenatal diagnosis can be performed once the diagnosis is confirmed and pathogenic mutations or variants are identified in previously affected children.[19,20] Genetic counseling is important before genetic testing and also if prenatal diagnosis is being contemplated in the family. Genetic counseling can be done by a certified genetic counselor, nurse counselor, or clinical geneticist. In autosomal recessive disorders, the risk of recurrence would be 25% and in X-linked disorders, the risk of recurrence would be 50% for males if the mutation is inherited in the family.
The sample for prenatal testing is taken at 10–14 weeks by chorionic villus sampling and at 16–22 weeks by amniocentesis. Sanger sequencing is done for the previously identified variant in affected child or carrier parents. The report is usually available in 1–2 weeks, and if the fetus is affected medical termination of pregnancy can be advised.
CONCLUSION
Many children with short stature are likely to have skeletal dysplasias. A good pattern recognition after evaluating the age of presentation, clinical clues, additional anomalies, and radiological findings helps in narrowing down the diagnostic possibilities. Molecular testing helps in the confirmation of diagnosis and facilitates prenatal diagnosis in selected families with severe phenotypes.
Acknowledgments
The authors thank Mrs. Rupinder Kaur for typographical assistance.
Declaration of patient consent
The authors certify that they have obtained all appropriate patient consent.
Conflicts of interest
There are no conflicts of interest.
Use of artificial intelligence (AI)-assisted technology for manuscript preparation
The author(s) confirm that there was no use of Artificial Intelligence (AI)-Assisted Technology for assisting in the writing or editing of the manuscript and no images were manipulated using AI.
Financial support and sponsorship
Nil.
References
- Nosology of genetic skeletal disorders: 2023 revision. Am J Med Genet A. 2023;191:1164-209.
- [CrossRef] [PubMed] [Google Scholar]
- Regulation of bone mass by Wnt signaling. J Clin Invest. 2006;116:1202-9.
- [CrossRef] [PubMed] [Google Scholar]
- Lethal and life-limiting skeletal dysplasias: Selected prenatal issues. Adv Clin Exp Med. 2021;30:641-7.
- [CrossRef] [PubMed] [Google Scholar]
- Frequency of skeletal dysplasia in children with short stature presenting to endocrine clinic: An observational study. J Family Med Prim Care. 2022;11:3143-7.
- [CrossRef] [PubMed] [Google Scholar]
- The birth prevalence rates for the skeletal dysplasias. J Med Genet. 1986;23:328-32.
- [CrossRef] [PubMed] [Google Scholar]
- Clinical trials in skeletal dysplasia: A paradigm for treating rare diseases. Br Med Bull. 2021;139:16-35.
- [CrossRef] [PubMed] [Google Scholar]
- Clinical management and emerging therapies of FGFR3-related skeletal dysplasia in childhood. Ann Pediatr Endocrinol Metab. 2022;27:90-7.
- [CrossRef] [PubMed] [Google Scholar]
- Clinical and genetic characteristics of COL2A1-related skeletal dysplasias in 60 Russian patients: Part I. Genes (Basel). 2022;13:137.
- [CrossRef] [PubMed] [Google Scholar]
- Over-representation of recessive osteogenesis imperfecta in Asian Indian children. J Pediatr Genet. 2020;11:81-6.
- [CrossRef] [PubMed] [Google Scholar]
- Standards and guidelines for the interpretation of sequence variants: A joint consensus recommendation of the American College of Medical Genetics and Genomics and the Association for Molecular Pathology. Genet Med. 2015;17:405-23.
- [CrossRef] [PubMed] [Google Scholar]
- Correction to: Diagnostic utility of next-generation sequencing-based panel testing in 543 patients with suspected skeletal dysplasia. Orphanet J Rare Dis. 2022;17:59.
- [CrossRef] [PubMed] [Google Scholar]
- WNT signaling perturbations underlie the genetic heterogeneity of Robinow syndrome. Am J Hum Genet. 2018;102:27-43.
- [CrossRef] [PubMed] [Google Scholar]
- Safe and persistent growth-promoting effects of vosoritide in children with achondroplasia: 2-year results from an open-label, phase 3 extension study. Genet Med. 2021;23:2443-7.
- [CrossRef] [PubMed] [Google Scholar]
- New perspectives on the treatment of skeletal dysplasia. Ther Adv Endocrinol Metab. 2020;11 doi: 10.1177/2042018820904016
- [CrossRef] [PubMed] [Google Scholar]
- Infigratinib in children with achondroplasia: The PROPEL and PROPEL 2 studies. Ther Adv Musculoskelet Dis. 2022;14 doi: 10.1177/1759720X221084848
- [CrossRef] [PubMed] [Google Scholar]
- Mucopolysaccharidosis IVA: Diagnosis, treatment, and management. Int J Mol Sci. 2020;21:1517.
- [CrossRef] [PubMed] [Google Scholar]
- Pharmacological interventions targeting pain in fibrous dysplasia/McCune-Albright syndrome. Int J Mol Sci. 2023;24:2550.
- [CrossRef] [PubMed] [Google Scholar]
- MATN3 mutation causing spondyloepimetaphyseal dysplasia. Indian J Pediatr. 2020;87:227-8.
- [CrossRef] [PubMed] [Google Scholar]
- Mutations in the CCN gene family member WISP3 cause progressive pseudorheumatoid dysplasia. Nat Genet. 1999;23:94-8.
- [CrossRef] [PubMed] [Google Scholar]