Translate this page into:
Comprehensive Overview of Congenital Adrenal Hyperplasia and its Genetic Diagnosis Among Children and Adolescents
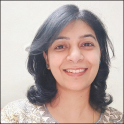
*Corresponding author: Sophy Korula, Former Professor, Department of Paediatrics, Paediatric Unit-1, Division of Paediatric Endocrinology, Christian Medical College and Hospital, Vellore, Tamil Nadu, India. jsophyhr@yahoo.co.in
-
Received: ,
Accepted: ,
How to cite this article: Korula S, Chapla A, Ravichandran L, George A. Comprehensive overview of congenital adrenal hyperplasia and its genetic diagnosis among children and adolescents. J Pediatr Endocrinol Diabetes 2022;2:119-30.
Abstract
The most common cause for congenital adrenal hyperplasia (CAH) is 21-hydroxylase deficiency (21OHD). This enzyme is encoded by the CYP21A2 gene which is highly homologous to its pseudogene CYP21A1P complicating the genetic analysis. We propose an algorithmic approach to genetic testing in 21OHD CAH utilizing multiple molecular techniques such as allele-specific polymerase chain reaction (ASPCR) and targeted gene sequencing to make it cost-effective. We recommend the classification of the common genetic mutations as Null and A–D, as established on functional enzyme studies. Pediatricians and pediatric endocrinologists need to be aware of the excellent genotypephenotype correlations established in the severe salt wasting CAH as highlighted in this review.
Keywords
Congenital adrenal hyperplasia
CAH
CYP21A2 gene
Genetics
21-hydroxylase deficiency
Pseudogene
Genetic counseling
CASE SCENARIOS
Case 1
A 34-day old baby presented with weight loss since birth, poor feeding, and decreased urine output for two days. He was referred from another hospital, and it was unclear what medications were given there. On examination, the baby appeared wasted with a weight loss of 19% since birth, and was hyperpigmented. Systemic examination was otherwise normal and genital examination showed bilaterally descended testes with hyperpigmented scrotum. Initial plasma sodium was 118 mmol/L, potassium 7.1 mmol/L, adrenocorticotropic hormone (ACTH) 104 pg/mL, and 17-hydroxyprogesterone (17OHP) was 144 ng/mL. A short synacthen test was performed which confirmed the diagnosis of congenital adrenal hyperplasia (CAH) based on suboptimal cortisol response and elevated 17OHP at baseline and post-stimulation [Table 1]. He was initiated on stress dose hydrocortisone and subsequently, fludrocortisone was added.
Biochemical parameter (Reference ranges) | 0 min | 30 min | 60 min |
---|---|---|---|
Cortisol (µg/dL)# | 17.19 | 18.2 | 19.4 |
17-hydroxyprogesterone (ng/mL)* (0.5–1.6) | 144.3 | 140.2 | |
Plasma renin activity | |||
(ng/mL/h) (4.4–46.1) | >60 | ||
Aldosterone | |||
(pg/mL) (40–310) | 3087 | ||
Dehydroepiandrosterone (µg/dL) (6–79) | 31.3 |
He was the second-born child to third-degree consanguineously married parents. At the most recent follow-up at 11 years and 8 months of age, he is doing well with levels of sodium 147 mmol/L, potassium 4.1 mmol/L, and 17OHP of 2.35 ng/mL while on oral hydrocortisone at a dose of 16.5 mg/m2/d and fludrocortisone at 50 µg once daily. His growth is optimal and his development is normal. He has not had any further adrenal crises and the family is aware of stress dosing. His elder sister is well and there was no family history of early neonatal deaths.
At nearly 9 years of age, he underwent in-house genetic testing as a part of our study on CAH genetic testing. A comprehensive approach with long-range polymerase chain reaction (PCR), restriction digestion, and allele-specific polymerase chain reaction (ASPCR) in this patient done in our laboratory indicated a large 30 kb deletion encompassing 3’ of CYP21A1P (pseudogene) and 5’ of CYP21A2 (functional gene) resulting in a classic chimeric deoxyribonucleic acid (DNA) (CH-8). This chimeric DNA carries multiple deleterious hotspot mutations in P30L, I2G, 8BPDEL, I172N, E6 CLUS, V281L, Q318X, and R356W in the homozygous state that leads to severe salt wasting phenotype. This was a unique case of eight homozygous mutations in the same patient. The case highlights the need for genetic testing and further genetic counseling as many families marry consanguineously or within close-knit communities in South India.
Case 2
A 27-year old primigravida who was diagnosed with CAH at 2.5 years of age presented for consultation after confirming her pregnancy at 6 weeks gestation. She was born to second-degree consanguineous parents. As a toddler, she was evaluated for failure to thrive with recurrent vomiting when she was noted to have clitoromegaly with normal blood pressure, and biochemical tests showed elevated 17OHP of 630 ng/mL (ref 0–1.1). Electrolytes were normal (sodium 135 mmol/L, and potassium 5.5 mmol/L) with normal plasma renin activity (PRA) 9.9 µIU/mL/h (ref 4.4–46.1). Serum dehydroepiandrosterone-sulfate (DHEAS) 21 µg/dL (ref 6–79) was also in the normal range. She was diagnosed with CAH at 2.5 years of age and initiated treatment on oral hydrocortisone and low dose fludrocortisone. Her weight centile improved dramatically on treatment, and she underwent a clitoral reduction surgery at 7 years of age. She had regular follow-up, attained menarche at 11.5 years of age and was otherwise well, attaining a final adult height of 154 cm. Her care was transitioned from pediatric to adult endocrinology services and she had good control throughout.
At this visit after confirming her pregnancy, a detailed discussion was held with the family and genetic testing was undertaken and her treatment was changed to dexamethasone. Her mother was homozygous positive for CYP21A2 c.518T>A (p.I172N) missense change which is classified as group B of CAH mutations with 2% enzyme activity. Her husband was a heterozygous carrier for 30 kb deletion carrying multiple pseudogene-derived mutations on one allele. The chorion villus sample (CVS) on the fetus done at 12 weeks of gestation confirmed the heterozygous carrier state of CYP21A2: c.518T>A (p.I172N) on one allele. The karyotype was 46,XX on the fetus [Figure 1]. After the availability of these reports, dexamethasone was changed back to hydrocortisone. The baby was well after birth and further genetic testing on the newborn sample confirmed the carrier status of the baby.

- Sanger chromatogram showing CYP21A2: c.518T>A (p.I172N) in homozygous state in the mother (proband) and heterozygous state in the fetus. CVS: Chorion villus sample.
INTRODUCTION
CAH comprises an extensive group of autosomal recessive disorders that result from a defect in the adrenal steroidogenesis pathway.[1] 21-Hydroxylase deficiency (21OHD) is the most common form of CAH and one of the most common inborn errors of metabolism. It is estimated that 90–99% of CAH results from this single enzyme deficiency which causes cortisol and aldosterone insufficiencies.[2,3] In this article 21OHD form of CAH alone is being discussed and hence used interchangeably with CAH. The clinical result of this deficiency is life-threatening salt wasting which needs to be identified early and managed effectively for a good outcome. The deficiency occurs from mutations in the CYP21A2 gene, the majority of which occur due to the unequal crossing over and gene conversion events between the functional CYP21A2 gene and non-functional CYP21A1P pseudogene. There are more than 230 pathogenic variants known to cause the biochemical deficiency posing a unique challenge in its genetic diagnosis.[4]
In this article, we will try and answer some of the common questions regarding CAH and aspects of its genetic diagnosis.
WHAT ARE THE COMMON FORMS OF 21OHD PRESENTING DURING CHILDHOOD AND ADOLESCENCE?
CAH from 21OHD is classified into three groups—classic salt wasting (SW-CAH), classic simple virilizing (SV-CAH), and non-classic (NC-CAH).[5] The combined estimated incidence of classic 21OHD is 1 in 14,000–18,000 live births, with approximately 75% classified as SW-CAH and 25% classified as SV-CAH. NC-CAH is more common than the classic forms, with an estimated incidence of 1 in 200.[6]
In SW-CAH, there is little or no residual enzymatic activity, resulting in extreme cortisol and aldosterone deficiencies. In addition, there is excess adrenal androgen synthesis as increased precursor steroids are diverted to the unaffected androgen pathways. If not recognized or treated, these infants present with “salt wasting crises” characterized by hyponatremia, hyperkalemia, acidosis, and shock.
The SV-CAH group with residual enzyme activity of 1–5% has slightly more aldosterone and hence is less likely to suffer acute salt wasting crisis. They present with varying degrees of virilization in girls and hyperandrogenic features in boys due to markedly elevated adrenal androgen production.[4] They also have cortisol deficiency necessitating treatment.[2] The genital appearance of affected 46,XX infants can give the appearance of male genitals with penis and scrotum but empty of gonads, an important clinical finding that confirms virilization. Müllerian duct formation is preserved although abnormality in urogenital sinus formation results in varying degrees of conjoined urethra and vagina. Thus, the reproductive potential exists in females despite atypical external genitalia.
In NC-CAH, the residual enzyme activity of 20–50% is noted resulting in late-onset or non-classical presentation. These children have normal basal cortisol and aldosterone production with mildly increased adrenal androgens. But nearly one-third still demonstrate suboptimal cortisol response on ACTH stimulation.[5] Children in this group present with symptoms from hyperandrogenism such as premature adrenarche, acne, and advanced skeletal maturation but many, especially males, are asymptomatic. Adolescent girls or adult women may present with hirsutism, oligomenorrhea, acne, and subnormal fertility.[5] The estimated prevalence of this milder type is nearly 1 in 200 in the Caucasian population.[6]
Table 2 summarizes the age-wise clinical pointers to a presumptive diagnosis of CAH.
Clinical pointers to 21OHD CAH ♦ Newborn and infancy •Salt wasting crises—weight loss, hyperpigmentation, hyponatremia, hyperkalemia, acidosis and shock. In girls, virilization of genitalia (varying degree as per Prader classification) is present. Often gets missed or diagnosis is delayed in boys •Virilization in girl newborns or infants ♦ Childhood •In early childhood can present with height spurt and acne in both girls and boys due to hyperandrogenism •Virilization in girls •Darkening of skin with virilization in girls •Behavioral abnormalities especially in boys with large penile size noted on examination with prepubertal testes—peripheral precocious puberty ♦ Adolescence •Short height compared to mid-parental height, with excessive acne or early change of voice in boys •Features of polycystic ovarian syndrome in girls—acne with increased facial hair in androgen dependent areas and oligomenorrhea •Hirsutism and premature adrenarche in girls •Rarely may present with thelarche and menarche noted in adolescents raised as boys (virilized 46,XX) |
CAH: Congenital adrenal hyperplasia, 21OHD: 21-Hydroxylase deficiency
WHAT DO WE NEED TO KNOW ABOUT NEWBORN SCREENING (NBS) FOR CAH?
Why is it needed?
It is well known that CAH is often missed to be diagnosed especially among male newborns. A retrospective analysis among sudden infant deaths confirmed 3/242 samples to have a genetic mutation positive for CAH.[7] Death rates ranging from 0–10% have been reported among SW-CAH.[8,9]
It is well established now that screening for CAH significantly decreases the time to diagnosis, severity of hyponatremia, and duration of hospitalization.[10,11] SW-CAH if diagnosed timely and treated early can prevent significant morbidity and mortality among neonates and infants.
What is the method is used for 17OHP measurement on NBS screen?
NBS for CAH was started with 17OHP measurement by immunoassay on the Guthrie card.[12] This was later replaced by automated time-resolved dissociation-enhanced lanthanide fluoroimmunoassay which is followed in most laboratories currently.
What are the factors that affect the level of 17OHP in newborns?
Several factors need to be considered when taking a decision based on 17OHP levels at NBS.
The level of 17OHP is high at birth and decline over the first few days in healthy infants, unlike in those with CAH where the levels increase with time.
Falsely high 17OHP levels are found when
17OHP levels are checked very early, especially in the first few days
Preterm and unwell neonates have higher levels of 17OHP as compared to term infants again resulting in false positives.
Interpretation of results needs to consider the poor specificity of immunoassays, especially regarding cut-off for low birth weight as quoted by most laboratories. Stratification based on gestational age rather than birth weight has better correlation and hence is more specific.[13]
The assay method is important to take note of, as prematurity with functional steroidogenic enzyme deficiency results in falsely high 17OHP values when using standard immunoassay due to cross reactivity with other steroid precursors.
One must also be aware of other rare forms of CAH which can have 17OHP elevation such as 11-hydroxylase deficiency, 3β-hydroxysteroid dehydrogenase deficiency, and P450 oxidoreductase deficiency.[14] The clinical presentation and electrolyte disturbances can be different in these and hence both the clinician and the lab must be aware of other diagnoses apart from 21OHD which can cause the same pathology.
Falsely low 17OHP levels may be seen with the following
Use of antenatal steroids can result in falsely low 17OHP levels in the newborn
Mild forms of 21OHD
Immature adrenal function with an immature biosynthetic pathway of cortisol can result in an inadequate 17OHP increase.
For the above reasons, it is advised to not collect NBS in the immediate postnatal period and to use liquid chromatography-tandem mass spectrometry (LC-MS/MS) for second-tier screening.[9] Up to 40% of samples on first-tier immunoassay screening show normal levels with LC-MS/MS method. If available, a short synacthen vis a vis a cosyntropin stimulation test should be performed to confirm the diagnosis. Few centers have adopted genetic testing at the second tier, and this remains to be established in the future. Endocrine Society Clinical Practice Guidelines comprehensively present these data for better understanding.[9]
What do we know from worldwide NBS data?
The first NBS screening was performed in Alaska in 1978 for 21OHD CAH and became mandatory in all 50 states by 2009.[15] The initial reports of poor positive predictive value (PPV) have been far improved in the recent past with better understanding of the time and method of testing. In 2014 data obtained over 26 years from Sweden reported 100% sensitivity for SW-CAH and 80% for SV-CAH.[16] The time of collection was after 48 h of life and gestational age-based cut-off values for 17OHP were used. The PPV of the screen was significantly higher than previous reports at 13.4% (25% for term and 1.4% for preterm babies). Data from Israel too showed a screening sensitivity of 95.4% for classic CAH (SWCAH and SV-CAH) with PPV of 16.5%.[17] Time of collection was between 36 and 72 h of life and 17OHP cut-off values were stratified by both gestational age and birth weight. The screening algorithm published from Brazil strongly discourages collection <48 h, after their data showed a PPV of 5.6% (46–72 h) and 14.1% (>72 h) based on birth weight adjusted 17OHP cut-off levels. One does need to balance the clear advantage of high PPV with delayed collection against the possibility of newborn presenting with a severe salt wasting crisis when NBS is done later, that is, 3–7 days and results remain pending, as noted in the Dutch study.[18]
Thus, it becomes clear that time adjusted cut-off values ensuring good PPV are needed with NBS for CAH and at the same time they should not be delayed so that salt wasting crises can be prevented.[3] The time frame of 48–72 h with gestational age-based cut-off values seems to be the most optimal option.
Regarding preterm infants, uniform guidelines are more difficult to establish with the consensus that 17OHP levels need monitoring at least 2–3 times within the first month usually at 2 weeks and 4 weeks of life to improve the accuracy of screening.[13-15]
WHAT IS THE DISEASE BURDEN OF CAH AND THE SCOPE OF NBS FOR CAH IN INDIA?
Although the exact disease burden of CAH in India is unclear, a recent study by the Indian Council of Medical Research (ICMR) Taskforce is suggestive of a significant burden in our country. In this large multicentric study, 18 of the 104,066 neonates screened were positive for CAH. There was a striking regional preponderance noted in South India with a 1 in 2036 prevalence as compared to 1 in 9983 in the western part of India.[19] Similar data have been confirmed by other Indian studies as well.[20] This could possibly be due to the high rate of consanguinity noted in South Indian population.
In our country, there are nearly 70,000 births every day with an annual birth rate of 17.3/1000 reported in 2021. CAH qualifies for newborn screening in Indian context as it is a prevalent disease with treatment available and timely treatment being able to prevent significant sequelae, fulfilling the Wilson and Jungner criteria. The practical and logistic difficulties with enormous births and significant number having home deliveries remains a huge challenge as highlighted extensively by Dabas et al.[21]
WHAT ARE THE DIFFERENT TESTS/ TECHNOLOGY AVAILABLE FOR GENETIC DIAGNOSIS OF CAH?
Although baseline and ACTH-stimulated 17OHP measurements are routinely used as the biochemical markers for CAH, these results are often unreliable with false positivity requiring secondary confirmation with LC-MS and so far, cannot specify whether an individual is a homozygote or heterozygote.[22] Therefore, genetic testing in CAH is highly advantageous to confirm the diagnosis of CAH and provide carrier screening. Genotyping CYP21A2 gene, implicated in 21OHD, the most common form of CAH is known to be extremely challenging due to a highly homologous pseudogene CYP21A1P present in proximity. Most of these mutations are pseudogene-derived due to meiotic crossing-over errors and gene conversion events. To overcome these challenges, several molecular techniques have been adapted in clinical and research setting.
The initial process is to amplify the functional gene without co-amplification of the pseudogene. This is usually achieved with long range PCRs for locus specific amplification of CYP21A2 gene that has consistently proven to be specific across several studies.[23,24] Following this, large 30 kb deletion and rearrangements can either be picked up with specifically designed long range PCRs or multiplex ligation-dependent probe amplification (MLPA). If these large deletions and rearrangements are ruled out, pseudogene-derived common mutations such as P30L, I2G, 8BPDEL, I172N, E6 CLUS, V281L, Q318X, and R356W can be identified [Figure 2] with genotyping techniques like ASPCR, that utilizes primer sets with 3’ terminal mismatches for wild type and mutant allele, restriction digestion with restriction enzyme specific for these mutations, real-time PCR based assays, and sequencing of the hotspot mutation regions. If negative for these hotspot mutations, sequencing the entire gene with Sanger or next generation sequencing (NGS) is carried out.

-
CYP21A2 gene with the most common pseudogene-derived point mutations. The exons are shown in dark grey boxes numbered from 1 to 10. The protein change of these mutations is marked on the exonic regions in the different zones of phenotypic severity that corresponds to the retained enzymatic activity depicted on the Y axis. The single nucleotide polymorphism database ID of these mutations is mentioned below the horizontal line. SW: Salt wasting, SV: Simple virilizing, NC: Non-classic.
The molecular complexity of CYP21A2 gene analysis is that one single technique cannot narrow down to the actual extent of mutation in 21OHD.[25,26] While long range PCRs are efficient in amplifying the functional gene and to rule out large rearrangements, genotyping techniques are limited to the hotspot mutations. MLPA used for gene deletions and duplications cannot provide the actual extent of chimeras that arise due to rearrangements between CYP21A2 and CYP21A1P gene.[27]
Several studies in India have been published regarding the molecular techniques used in CAH diagnosis as highlighted in Table 3.[28-33] At Christian Medical College and Hospital, Vellore, we used comprehensive approach ASPCR followed by NGS in those affordable or negative on the ASPCR.[28] This method helped diminish costs tremendously such that we are currently offering the ASPCR for Rs. 2500 (INR) only.
Study (ref) | Molecular techniques | Genes analyzed | Diagnostic yield (%) (sample size) | Predominant mutation in CYP21A2 gene |
---|---|---|---|---|
Mathur et al., 2001[29] | ASPCR for 6 hotspots | CYP21A2 | 78 (n=28) | I172N |
Marumudi et al., 2012[30] | Genotyping with restriction digestion | CYP21A2 | 74.2 (n=62) | I2G |
Yadav et al., 2015[31] | Genotyping with restriction digestion | CYP21A2 | 100 (n=13) | P30L |
Khajuria et al., 2017[32] | Nested PCR, SSCP, RFLP | CYP21A2 | 96.4 (n=55) | I2G |
Gangodkar et al., 2021[33] | NGS | CYP21A2 | 82.6 (n=310) | I2G |
Ravichandran et al., 2021[34] | ASPCR, NGS | CYP21A2, CYP11B1, CYP17A1, CYP19A1, POR | 97.2 (n=72) | I2G |
CAH: Congenital adrenal hyperplasia, PCR: Polymerase chain reaction, NGS: Next generation sequencing, ASPCR: Allele-specific polymerase chain reaction, SSCP: Single-strand confirmation polymorphism analysis, RFLP: Restriction fragment length polymorphism
On identification, understanding the extent and severity of these mutations is required to correlate clinically and optimize therapeutic measures. Based on this experience and published data with excellent diagnostic yield, we would recommend a stepwise comprehensive approach with multiple techniques to delineate the underlying genetic cause and enable effective family screening [Figure 3]. A combination of long-range PCR with one of the genotyping assays can identify large rearrangements and point mutations along with specific chimeras. On the other hand, a primary requisite for NGS analysis of CYP21A2 gene is the development of computational pipelines with stringent filters that will restrict alignment of the reads to the reference region of CYP21A2 gene and not CYP21A1P gene.

- Algorithmic representation of genetic tests that can be applied in a stepwise manner for comprehensive and cost-effective genetic diagnosis of congenital adrenal hyperplasia.
HOW DO WE CHOOSE A SPECIFIC GENETIC TEST/TECHNIQUE FOR A GIVEN CHILD WITH SUSPECTED GENETIC FORM OF CAH?
Based on the clinical suspicion and biochemical findings, if the subject presents with a classical salt wasting or simple virilizing form, the first step is to identify pseudogene-derived long rearrangements and point mutations. 85–95% of the subjects can be genetically confirmed of 21OHD at this point. This is also cost effective and less time consuming in a developing country like India where many can still not afford single or multigene sequencing for CAH.
If negative, proceed with sequencing the complete CYP21A2 gene. For subjects with non-classic forms of CAH, or subjects with other forms of CAH, followed by the first step, a targeted NGS panel of the most implicated genes— CYP11A1, CYP11B1, CYP17A1, HSD3B2, POR and StAR can be utilized. If found negative for these genes, a clinical exome or a whole exome sequencing can be considered. The flow chart explains the proposed detailed algorithm of CAH genetic testing that has been in use in our clinical setting that has proven to be comprehensive and cost effective for Indian scenario [Figure 3].
IS THERE A SPECIFIC GENOTYPE-PHENOTYPE CORRELATION WITH VARIOUS GENETIC FORMS KNOWN SO FAR?
CAH being an autosomal recessive disorder, the phenotype severity is dictated by the allele carrying the least severe mutation. Typically, we classify CAH to be classic (either SWCAH or SV-CAH) and NC-CAH. We know that complete inactivation results in SW-CAH phenotype, enzyme activity of around 2% causes SV-CAH phenotype and activity between 10% and 75% results in NC-CAH phenotype.[34]
Excellent work by New et al. on a heterogenous population brought in a lot of light to the genotype-phenotype correlations in CAH.[35] By computational modeling humanizing the crystal structure of bovine CYP21A2 was established and it was noted that mutations affecting critical enzyme functions like membrane anchoring, heme binding, substrate binding, or that alter enzyme stability caused a complete non-functionality of the enzyme resulting in SW-CAH. On the other hand, mutations that affected the transmembrane region or conserved hydrophobic patches resulted in about a 98% reduction in enzyme activity and caused SV-CAH. Alteration in oxidoreductase interactions, salt-bridge and hydrogen bonding networks, and nonconserved hydrophobic clusters resulted in the milder NCCAH. This data established genotype-phenotype correlation comprehensively and has been highlighted in the text box below [Box 1].
Further, well established in vivo studies have described the effect of these pseudogene-derived hotspot mutations.[36] Based on the extent of loss of the enzyme activity, the mutations have been classified into four groups.[37]
The Null group involving large deletions, gene conversion, point mutations such as E6 cluster in exon 6 (I235N, V236E, and M238K), 8 bp deletion in exon 3, p.Gln319Ter (Q318X) and p.Arg357Trp (R356W) in exon 8 result in zero enzyme activity leading to SWCAH phenotype
Group A consists of the intron 2 splice mutation (I2G) that results in 0–1% of the enzyme activity also expected to result in SW-CAH phenotype
Group B with p.Ile173Asn (I172N) in exon 4 results in 1–2% of the enzyme activity usually result in SV-CAH phenotype
Group C consists of p.Pro31Leu (P30L) in exon 1, p.Val282Leu (V281L) in exon 8 and p.Pro454Ser (P453S) in exon 10 with 20–60% enzyme activity and lead to NCCAH phenotype.
Around 90% of the cases show good genotype-phenotype correlation in 21OHD.
Although the nature of these mutations has been well described, there exists a genotype-phenotype discordance in some of the cases in particular to I2G and I172N mutations that alternate phenotypes between SW-CAH and SV-CAH.[26] P30L, on the other hand, has been reported to result in SV-CAH forms in few cases. For the other non-pseudogene-derived mutations (>230 in HGMD database) establishing clear genotype-phenotype correlations is an enormous task often requiring molecular modeling functional investigations.
WHICH ARE THE STANDARD LABORATORIES OFFERING GENETIC DIAGNOSIS OF GENETIC FORMS OF CAH IN INDIA? WHAT IS THE APPROXIMATE COST?
Several common private labs offer genetic testing of CAH, the names have been kept anonymous for the purpose of this article. The approximate cost of the genetic tests for the diagnosis of CAH is given in [Table 4].
Lab | Test offered | Cost | Turnaround time |
---|---|---|---|
A | Deletion/duplication analysis by MLPA | Rs. 12,000 | 21 days |
B | NGS+MLPA | Rs. 12,000 | 21 days |
C | MLPA+Sanger sequencing | Rs. 5,000 | 21 days |
D | 8 gene panel with Sanger confirmation+CNV analysis with MLPA/QPCR confirmation+CYP21A2 analysis | Rs. 36,000 | 30 days |
CAH: Congenital adrenal hyperplasia, NGS: Next generation sequencing, MLPA: Multiplex ligation-dependent probe amplification, CNV: Copy number variation, QPCR: Quantitative polymerase chain reaction
Institute of Genomics and Integrative Biology, New Delhi, Centre for Human Genetics, Bangalore, Institute of Medical Genetics at Sir Gangaram Hospital, New Delhi and Christian Medical College, Vellore, are few of the other leading organizations offering genetic testing for congenital adrenal hyperplasia. In CMC Vellore, ASPCR for the common 8 mutations is offered at Rs. 2500 (INR).
HOW DO WE COUNSEL THE FAMILY WHEN A CHILD IS DIAGNOSED WITH A GENETIC FORM OF CAH?
Genetic counseling should be provided to parents at the time of birth of a child with CAH as well as adolescents at the time of transition to adult care, according to the Endocrine Society Clinical Practice Guidelines.[38]
Heterozygotes cannot be distinguished from the general population using biochemical assays. Thus, genotyping is helpful for genetic counseling and is a useful tool for determining the carrier status in an individual.[35]
Several crucial aspects should be considered when providing genetic counseling:
Genetic testing should be performed in a reputable lab and reports analyzed by a qualified professional
Pre-conception period is the optimal time for determination of the genetic risk, carrier status, or treatment
Testing of reproductive partners of probands for carrier status is recommended when one or both disease-causing mutations have been identified in the proband
Before testing, caregivers should be fully informed about the risks and benefits. They should be aware of the prognosis, morbidity, mortality, and potential effects of a positive genetic screen on the patient or their family.[39]
Counseling of children
Both parents as well as physicians should inform the child about his/her disease. The information should be given in a way appropriate for the child’s age and developmental stage, and it should be repeated at regular intervals, beginning at a young age. Adolescents should be reassured and, if necessary, offered individual counseling sessions.[40] Early involvement of a multidisciplinary team, comprising of a pediatrician, geneticist, pediatric surgeon, and child psychiatrist is crucial.
Risk calculation
Successive siblings of a child affected with CAH have a 25% chance of developing CAH, and 50% chance of being an asymptomatic carrier for this autosomal recessive condition.[38] About two-third of patients with NC-CAH are compound heterozygotes, meaning that they possess both the alleles causing classic CAH and NC-CAH. If the carrier status of the spouse is unknown, the likelihood that a patient with NC-CAH will have a child with classic CAH is 1 in 360.[40]
Patient counseling model
A successful patient counseling model would incorporate patients’ values and attitudes regarding their illness. The risks and benefits of genetic screening should be stressed. A complete personal medical and family history (including a detailed family pedigree), information regarding the genetics of the ailment, and conversations on prevention and screening alternatives are further elements of an effective counseling model.[39]
Fertility counseling
It is recommended that a patient with CAH and infertility be referred to a reproductive endocrinologist and/or fertility specialist. Fertility is impaired in males with CAH due to various reasons such as presence of testicular adrenal rest tumors (TARTs), testicular failure and suppression of gonadotropins. TARTs usually respond to intensified glucocorticoid therapy. Testicular sperm extraction and sperm banking are also helpful in maintaining fertility. By optimizing glucocorticoid and mineralocorticoid therapy and thereby adequately suppressing follicular phase progesterone, women with CAH can attain near normal pregnancy rates.[38]
WHAT IS THE ROLE OF GENETIC DIAGNOSIS IN PREMARITAL, PRE-PREGNANCY, ANTENATAL, AND PERINATAL GENETIC COUNSELING?
Premarital
It is prudent to offer molecular testing to the reproductive partner of an affected individual since there is a high carrier rate for 21OHD
The child’s risk of developing CAH is significantly reduced if the reproductive partner is not a carrier
However, each child has a 50% chance of being afflicted if the reproductive partner is discovered to be heterozygous for a known pathogenic variation.[41]
Pre-pregnancy
Pre-pregnancy is the ideal time to determine genetic risk, clarify carrier status, and discuss the availability of prenatal/preimplantation genetic testing
It is appropriate to offer genetic counseling to young adults who are affected, are carriers, or are at risk of being carriers
Discussion of potential risks to offspring and reproductive options are essential.[41]
Antenatal and perinatal
In high-risk pregnancies, we can antenatally determine if the fetus has CAH by molecular genetic testing. Understanding the phenotype before birth can enable quicker and more targeted treatment
Yau et al. reported a strategy to avoid unwarranted prenatal dexamethasone treatment of male and unaffected female fetuses by utilizing non-invasive prenatal testing for CAH using targeted massive parallel sequencing on fetal cell-free DNA in maternal plasma[42]
In low-risk pregnancies, fetal genital or adrenal abnormalities can be detected on prenatal ultrasonography[43]
A fetal karyotype, fluorescence in situ hybridization (FISH) for SRY (sex determining region of Y protein), and ultrasound evaluation for Müllerian structures should be obtained if ambiguous external genitalia are discovered during an ultrasound examination. Amniocentesis or CVS for molecular genetic testing of CYP21A2 may be appropriate[41]
Preimplantation genetic testing and the transfer of unaffected embryos would prevent the need to treat an unaffected embryo.[44]
TREATMENT OF GENETIC FORMS OF CAH – DOES IT MAKE A DIFFERENCE?
Specific genotypes have been proven to be associated with SW, SV, or NC forms of CAH, with an excellent genotypephenotype correlation. With less severe disease, the genotype-phenotype link becomes less significant.[45]
Gene deletions and large conversions—8 bp deletion, E6 cluster, p.Gln319Ter (Q318X) and p.Arg357Trp (R356W) are commonly associated with classic SW-CAH, the most severe form of the disease, warranting treatment with glucocorticoids and fludrocortisone. Missense pathogenic variant I172N (p.[Ile173Asn]) confers around 1–2% 21-hydroxylase activity. This produces nearly normal aldosterone production, thereby leading to the SV-CAH necessitating only glucocorticoid supplementation. p.Pro31Leu (P30L), p.Val282Leu (V281L) and p.Pro454Ser (P453S) are pathogenic variants commonly associated with NC-CAH.[46]
Therefore, CYP21A2 genotyping of the fetus is predictive of his/her clinical outcome. As a result, many institutions provide CYP21A2 genotyping in conjunction with prenatal diagnosis and dexamethasone therapy.[45] Dexamethasone is given to pregnant woman beginning in the 6th–7th week of gestation as part of the prenatal therapy of this illness to ameliorate the symptoms of virilization in affected female fetuses.[47] However, it is crucial to note that prenatal therapy is still viewed as experimental because of conflicting information, notably concerning the treatment’s outcome and the safety of both mothers and their unborn children.[45] Since the discovery of cell-free fetal DNA in the mother’s bloodstream, it is now possible to identify the gender of the fetus by the seventh week of pregnancy. Thus, adopting this novel technique could potentially prevent treating male babies and healthy female fetuses while partially addressing some ethical issues with prenatal therapy.[39] Preimplantation genetic testing in conjunction with in vitro fertilization techniques have been attempted in at-risk pregnancies. Only genetically transferable embryos — those free of the disease — are transferred to the woman’s uterus after molecular genotyping and haplotyping procedures. This limits the spread of a disease within a family.[44]
Many of these have internal ethical concerns and the scope of this paper does not allow to address that. The authors have highlighted the possibilities contextually and do not confirm to the use of genetically transferrable embryos. Antenatal gender identity again will have legal bearing based on the country of residence and hence the experimental dexamethasone therapy remains as a decision made on a case-to-case basis by an experienced endocrinologist after detailed discussion with family and considering the local practices and rules.
CONCLUSION
There is significant burden of CAH in India as highlighted by the recent newborn screening data published by the ICMR Taskforce
Genetic testing in 21OHD is challenging and complex due to a highly homologous pseudogene CYP21A1P present near the to the CYP21A2 gene
An algorithmic approach to genetic testing utilizing multiple molecular techniques as highlighted in Figure 2 will help in comprehensive and cost-effective analysis, a felt need in our country
The common genetic mutations can be classified as Null and A-D based on functional enzyme studies. This awareness will help clinicians tailor therapy accordingly
Majority of the mutations in the severe group SW-CAH show excellent genotype-phenotype correlation
Genetics do not depose biochemical testing, rather add an additional benefit of phenotypic prediction, and assist with genetic counseling.
Acknowledgments
The authors would like to acknowledge Dr. Nihal Thomas, Professor, Department of Endocrinology and Diabetes and Dr Swathi Rathore, Professor, Obstretics and Gynaecology, Christian Medical College Hospital, Vellore, for clinical and antenatal care of case 2.
Declaration of patient consent
Patient’s consent not required as patients identity is not disclosed or compromised.
Conflicts of interest
There are no conflicts of interest.
Financial support and sponsorship
Nil.
References
- The molecular biology, biochemistry, and physiology of human steroidogenesis and its disorders. Endocr Rev. 2011;32:81-151.
- [CrossRef] [PubMed] [Google Scholar]
- Congenital adrenal hyperplasia-current insights in pathophysiology, diagnostics, and management. Endocr Rev. 2022;43:91-159.
- [CrossRef] [PubMed] [Google Scholar]
- Newborn screening for congenital adrenal hyperplasia: Review of factors affecting screening accuracy. Int J Neonatal Screen. 2020;6:67.
- [CrossRef] [PubMed] [Google Scholar]
- Congenital adrenal hyperplasia (CAH) due to 21-hydroxylase deficiency: A comprehensive focus on 233 pathogenic variants of CYP21A2 gene. Mol Diagn Ther. 2018;22:261-80.
- [CrossRef] [PubMed] [Google Scholar]
- Nonclassic congenital adrenal hyperplasia due to 21-hydroxylase deficiency: Clinical presentation, diagnosis, treatment, and outcome. Endocrine. 2015;50:32-50.
- [CrossRef] [PubMed] [Google Scholar]
- Revisiting the prevalence of nonclassic congenital adrenal hyperplasia in US Ashkenazi Jews and Caucasians. Genet Med. 2017;19:1276-9.
- [CrossRef] [PubMed] [Google Scholar]
- Prevalence of congenital adrenal hyperplasia among sudden infant death in the Czech Republic and Austria. Eur J Pediatr. 2007;166:1-4.
- [CrossRef] [PubMed] [Google Scholar]
- Newborn screening: Toward a uniform screening panel and system. Genet Med. 2006;8(Suppl 1):1S-252.
- [Google Scholar]
- How many deaths can be prevented by newborn screening for congenital adrenal hyperplasia? Horm Res Paediatr. 2007;67:284-91.
- [CrossRef] [PubMed] [Google Scholar]
- Effect of newborn screening for congenital adrenal hyperplasia. Arch Pediatr Adolesc Med. 1999;153:1272-8.
- [CrossRef] [PubMed] [Google Scholar]
- Benefits of neonatal screening for congenital adrenal hyperplasia (21-hydroxylase deficiency) in Sweden. Pediatrics. 1998;101:E11.
- [CrossRef] [PubMed] [Google Scholar]
- Comprehensive cost-utility analysis of newborn screening strategies. Pediatrics. 2006;117:S287-95.
- [CrossRef] [PubMed] [Google Scholar]
- Cutoff levels of 17-alpha-hydroxyprogesterone in neonatal screening for congenital adrenal hyperplasia should be based on gestational age rather than on birth weight. J Clin Endocrinol Metab. 2005;90:3904-7.
- [CrossRef] [PubMed] [Google Scholar]
- Congenital adrenal hyperplasia: Time to replace 17OHP with 21-deoxycortisol. Horm Res Paediatr. 2019;91:416-20.
- [CrossRef] [PubMed] [Google Scholar]
- Newborn screening for congenital adrenal hyperplasia with special reference to screening in Alaska. Ann N Y Acad Sci. 1985;458:90-102.
- [CrossRef] [PubMed] [Google Scholar]
- Nationwide neonatal screening for congenital adrenal hyperplasia in Sweden: A 26-year longitudinal prospective population-based study. JAMA Pediatr. 2014;168:567-74.
- [CrossRef] [PubMed] [Google Scholar]
- Combined gestational age-and birth weight-adjusted cutoffs for newborn screening of congenital adrenal hyperplasia. J Clin Endocrinol Metab. 2019;104:3172-80.
- [CrossRef] [PubMed] [Google Scholar]
- Evaluation of the Dutch neonatal screening for congenital adrenal hyperplasia. Arch Dis Child. 2019;104:653-7.
- [CrossRef] [PubMed] [Google Scholar]
- Newborn screening for congenital hypothyroidism and congenital adrenal hyperplasia. Indian J Pediatr. 2018;85:935-40.
- [CrossRef] [PubMed] [Google Scholar]
- Newborn screening for congenital adrenal hyperplasia in India: What do we need to watch out for? J Obstet Gynaecol India. 2016;66:415-9.
- [CrossRef] [PubMed] [Google Scholar]
- CAH newborn screening in India: Challenges and opportunities. Int J Neonatal Screen. 2020;6:70.
- [CrossRef] [PubMed] [Google Scholar]
- Recent advances in biochemical and molecular analysis of congenital adrenal hyperplasia due to 21-hydroxylase deficiency. Ann Pediatr Endocrinol Metab. 2016;21:1-6.
- [CrossRef] [PubMed] [Google Scholar]
- Novel method to characterize CYP21A2 in Florida patients with congenital adrenal hyperplasia and commercially available cell lines. Mol Genet Metab Rep. 2014;1:312-23.
- [CrossRef] [PubMed] [Google Scholar]
- Mutational analysis of CYP21A2 gene and CYP21A1P pseudogene: Long-range PCR on genomic DNA. Methods Mol Biol. 2014;1167:275-87.
- [CrossRef] [PubMed] [Google Scholar]
- Comprehensive mutation analysis of the CYP21A2 gene: An efficient multistep approach to the molecular diagnosis of congenital adrenal hyperplasia. J Mol Diagn. 2013;15:745-53.
- [CrossRef] [PubMed] [Google Scholar]
- Genetics of congenital adrenal hyperplasia. Best Pract Res Clin Endocrinol Metab. 2009;23:181-92.
- [CrossRef] [PubMed] [Google Scholar]
- Allele-specific and multiplex PCR based tools for cost-effective and comprehensive genetic testing in congenital adrenal hyperplasia. MethodsX. 2022;9:101748.
- [CrossRef] [PubMed] [Google Scholar]
- Allele-specific PCR and next-generation sequencing based genetic screening for congenital adrenal hyperplasia in India. Eur J Med Genet. 2021;64:104369.
- [CrossRef] [PubMed] [Google Scholar]
- Molecular characterization of mutations in Indian children with congenital adrenal hyperplasia due to steroid 21-hydroxylase deficiency. J Pediatr Endocrinol Metab. 2001;14:27-35.
- [CrossRef] [PubMed] [Google Scholar]
- Molecular genetic analysis of CYP21A2 gene in patients with congenital adrenal hyperplasia. Indian J Endocrinol Metab. 2012;16:384-8.
- [CrossRef] [PubMed] [Google Scholar]
- Clinical profile and inheritance pattern of CYP21A2 gene mutations in patients with classical congenital adrenal hyperplasia from 10 families. Indian J Endocrinol Metab. 2015;19:644-8.
- [CrossRef] [PubMed] [Google Scholar]
- The spectrum of CYP21A2 mutations in Congenital Adrenal Hyperplasia in an Indian cohort. Clin Chim Acta Int J Clin Chem. 2017;464:189-94.
- [CrossRef] [PubMed] [Google Scholar]
- Clinical application of a novel next generation sequencing assay for CYP21A2 gene in 310 cases of 21-hydroxylase congenital adrenal hyperplasia from India. Endocrine. 2021;71:189-98.
- [CrossRef] [PubMed] [Google Scholar]
- Inhibition of CYP21A2 enzyme activity caused by novel missense mutations identified in Brazilian and Scandinavian patients. J Clin Endocrinol Metab. 2008;93:2416-20.
- [CrossRef] [PubMed] [Google Scholar]
- Genotype-phenotype correlation in 1,507 families with congenital adrenal hyperplasia owing to 21-hydroxylase deficiency. Proc Natl Acad Sci U S A. 2013;110:2611-6.
- [CrossRef] [PubMed] [Google Scholar]
- Congenital adrenal hyperplasia due to steroid 21-hydroxylase deficiency. Clin Endocrinol (Oxf). 1998;49:411-7.
- [CrossRef] [PubMed] [Google Scholar]
- Genetics of congenital adrenal hyperplasia. Best Pract Res Clin Endocrinol Metab. 2009;23:181-92.
- [CrossRef] [PubMed] [Google Scholar]
- Congenital adrenal hyperplasia due to steroid 21-hydroxylase deficiency: An endocrine society clinical practice guideline. J Clin Endocrinol Metab. 2018;103:4043-88.
- [CrossRef] [PubMed] [Google Scholar]
- Genetics of congenital adrenal hyperplasia. Endocrinol Metab Clin North Am. 2017;46:435-58.
- [CrossRef] [PubMed] [Google Scholar]
- Management of adolescents with congenital adrenal hyperplasia. Lancet Diabetes Endocrinol. 2013;1:341-52.
- [CrossRef] [PubMed] [Google Scholar]
- 21-hydroxylase-deficient congenital adrenal hyperplasia. In: Adam MP, Everman DB, Mirzaa GM, Pagon RA, Wallace SE, Bean LJ, eds. GeneReviews®. Seattle, WA: University of Washington, Seattle; 1993.
- [Google Scholar]
- Prenatal diagnosis of congenital adrenal hyperplasia owing to 21-hydroxylase deficiency. Expert Opin Orphan Drugs. 2015;3:487-90.
- [CrossRef] [Google Scholar]
- Sonography in prenatal diagnosis of congenital adrenal hyperplasia. Prenat Diagn. 2004;24:627-30.
- [CrossRef] [PubMed] [Google Scholar]
- Prenatal genetic testing and treatment for congenital adrenal hyperplasia. Fertil Steril. 2019;111:21-3.
- [CrossRef] [PubMed] [Google Scholar]
- EMQN best practice guidelines for molecular genetic testing and reporting of 21-hydroxylase deficiency. Eur J Hum Genet. 2020;28:1341-67.
- [CrossRef] [PubMed] [Google Scholar]
- Congenital adrenal hyperplasia-the main effect of 21-hydroxylase deficiency In: Adrenal Glands-the Current Stage and New Perspectives of Diseases and Treatment. London, UK: IntechOpen; 2022. Available from: https://www.intechopen.com/state.item.id [Last accessed on 2022 Dec 29]
- [CrossRef] [Google Scholar]
- Prenatal dexamethasone treatment in pregnancies at risk for congenital adrenal hyperplasia due to 21-hydroxylase deficiency: Effect on midgestational amniotic fluid steroid levels. J Clin Endocrinol Metab. 1993;76:117-20.
- [CrossRef] [PubMed] [Google Scholar]